Nur Schettino ‘24 and Professor Fe McBride search for Sources of Cosmic Neutrinos
By Sean Li '23South Pole Stargazing
Far beneath the South Pole are buried thousands of spherical light sensors in a lattice that takes up a cubic kilometer of pure Antarctic ice. These sensors belong to the IceCube Neutrino Observatory, a collaboration that looks for the nearly massless, uncharged subatomic particles in its name. Due to their minimal mass and lack of charge, neutrinos seldom interact with matter, earning them the moniker “ghost particles.”
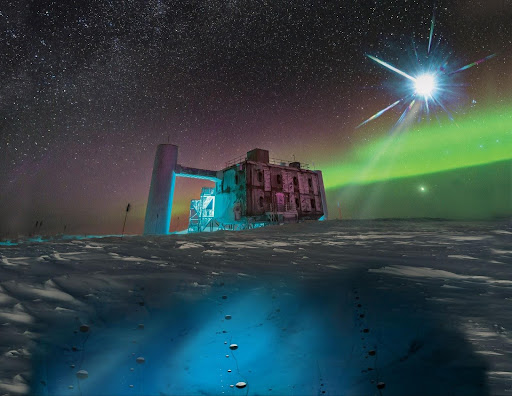
Cosmic neutrinos, so-called because they come from astrophysical objects in outer space, can arrive at Earth with energies in the hundreds of TeV (tera electron Volts, or 1012 eV) up to one PeV (peta electron Volts, or a thousand TeV). When a high-energy cosmic neutrino strikes a water molecule in the IceCube detector, it can discharge an electron that moves faster than light does in ice. The luminous equivalent of a sonic boom ensues; this same phenomenon, known as Cherenkov radiation, also occurs in nuclear reactors submerged in water, and the human eye perceives it as a blue glow. This animation illustrates what happens.
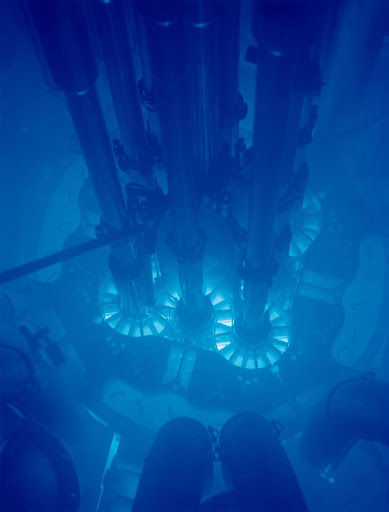
The blue glow of Cherenkov radiation in the Advanced Test Reactor at the Idaho National Laboratory. Credit: DOE.
Using its many sensors to record the light cone of the Cherenkov radiation, IceCube can reconstruct the trajectory of the responsible neutrino, narrowing down its origin in the sky. Since neutrinos are electrically neutral, they travel in straight lines from their sources to the detector, eliminating possibilities like a neutrino “pirouetting,” as Schettino words it, through the atmosphere due to Earth’s magnetic field. The cosmic neutrinos that reach Earth come from the interactions of charged particles in outer space. The big question that excites Schettino is: What astrophysical events produce the neutrinos that IceCube detects?
Black-Hole Brilliance
The extreme gravity of a supermassive black hole, like the one at the center of our galaxy, can accelerate ions to such high speeds that neutrinos emerge out of their collisions. These black holes, which can devour nearby stars, attract gas and dust that assembles into a rapidly spinning accretion disk whose electromagnetic (EM) radiation outshines the rest of the galaxy. For that reason, they’re identified as active galactic nuclei, or AGN.
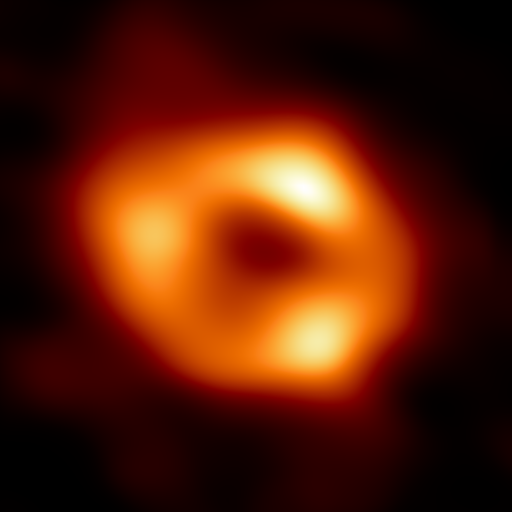
The shadow of Sagittarius A*, the supermassive black hole at the center of the Milky Way Galaxy. The Event Horizon Telescope (EHT) Collaboration revealed this composite image to the world in May 2022.
Schettino says that understanding AGN as neutrino sources could help explain how heavy nuclei, like iron, gain the enormous kinetic energy required to reach Earth at relativistic speeds, which continues to puzzle astronomers.
In their research, Schettino and McBride focused on a specific high-energy neutrino that IceCube detected. Schettino explains, “IceCube gives us a region, and then we search the astronomical catalogs for candidate sources in that region.”
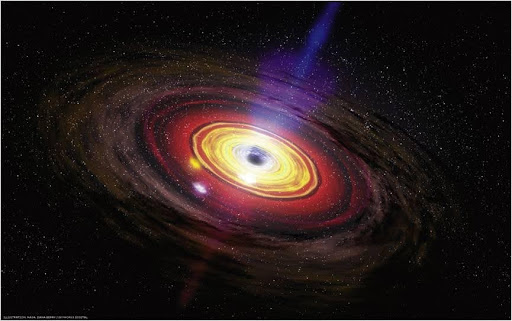
An illustration of an active galactic nucleus with an accretion disk and a high-energy jet along its rotation axis. Such AGN, Schettino says, could accelerate charged particles and eject high-energy neutrinos. Credit: NASA/Dana Berry (SkyWorks Digital).
Coding & Calculating
To assess candidate neutrino sources, Schettino needed to analyze a lot of data. He used a scripting language called S-Lang and the Interactive Spectral Interpretation System, a package of data analysis functions designed for studying X-ray spectra.
Why X-rays? “Ostensibly, EM data have nothing to do with neutrinos,” says Schettino. But in 2000, researchers presented an astrophysical simulator whose results show that the energy flux of neutrinos and photons in extreme environments like AGN are very similar. For that reason, one can estimate the number of neutrinos emitted in a given time interval using the X-ray energy flux for that same interval.
To calculate the X-ray energy flux from candidate sources, Schettino constructed spectral energy distribution (SED) curves by plotting energy flux against photon frequency.
“There are many parameters to consider, such as the curvature, slope, and range of these SEDs; playing around with them allows us to see how well we can fit the data,” explains Schettino.
Surprisingly, the SED data for many sources can be modeled using parabolas on a log-log scale. In particular, to model AGN spectra, researchers employ a pair of 2 parabolas that somewhat resemble the humps of a camel, earning these SED curves the nickname “camel plots.”
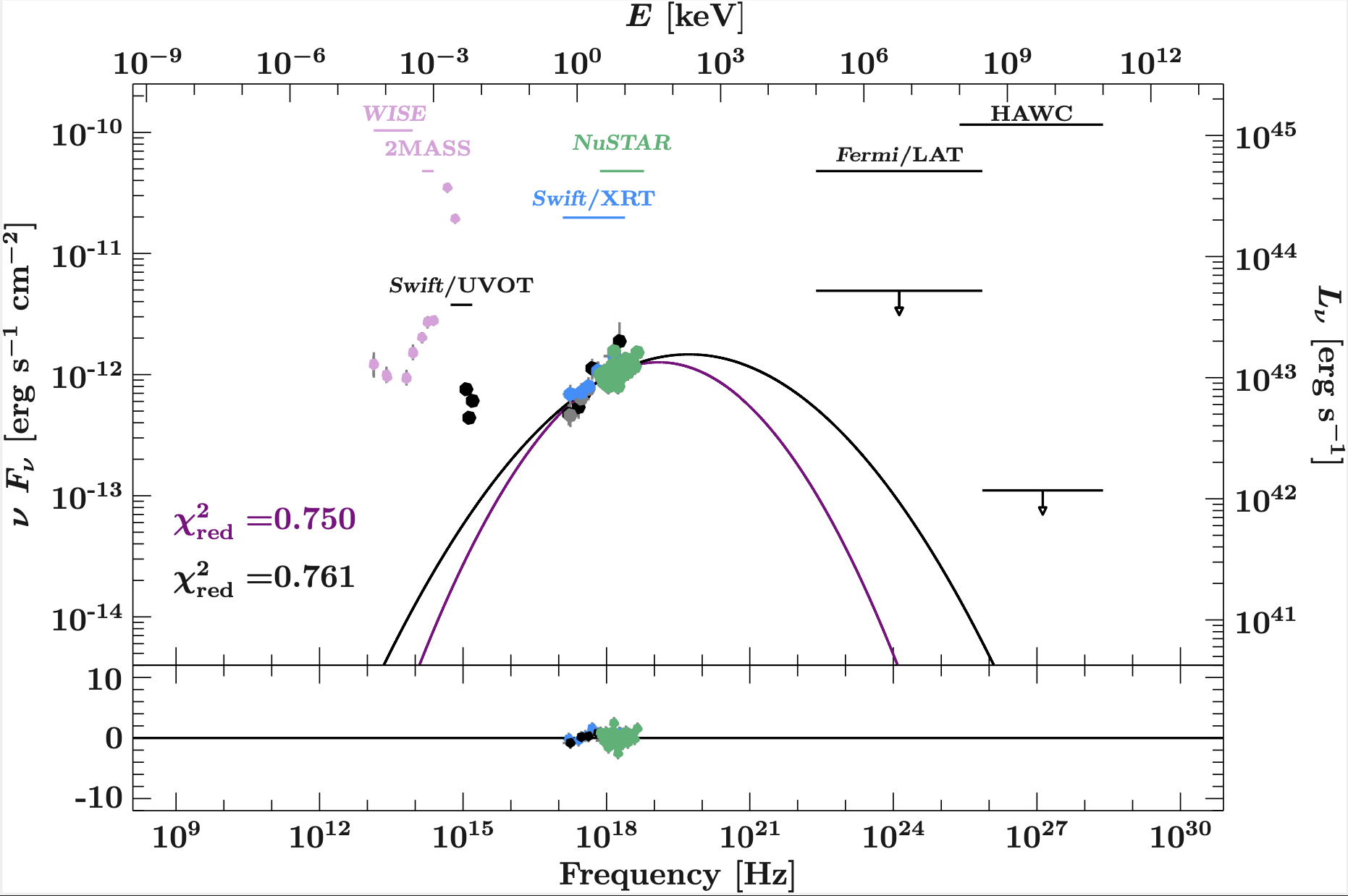
An example of a “camel plot” courtesy of Schettino (in-progress)
“For the neutrino event that we considered, the only real candidate source is an AGN,” Schettino explains.
By integrating over the SED, Schettino calculated the photon energy flux from a candidate AGN and used that to predict the corresponding neutrino energy flux. Then, using a key assumption (namely, that the higher-energy parabola comes from “purely hadronic” interactions involving protons, pions, or any other particles made of quarks), accounting for the limits of IceCube’s sensitivity, and adjusting for effects like absorption by stellar dust, Schettino was able to obtain a neutrino number. In essence, the neutrino number represents how many neutrinos IceCube would expect to detect in a given time interval.
“Say, for example, we calculate a neutrino number of 0.5 for a given time period, but IceCube actually detected one neutrino in that period. Then we would use Poisson statistics,” a type of low-number statistics, “to calculate the probability of that one detection.”
Schettino and McBride are now preparing a manuscript to present their results.
A Stellar Summer
This work fascinates Schettino. For one thing, he says, “IceCube is such an incredibly cool project; it’s a marvel of engineering.” And he’s passionate about astrophysics, especially involving extreme gravity: “I love black holes.”
On the technical side of the project, Schettino reflects, “I loved thinking about how physics informed decisions in the code. It’s easy to get lost in the details of script-writing, but the model needs to represent reality.” For example, he explains, “When fitting a power law to X-ray data, we have to apply an absorption model because EM radiation gets absorbed by cosmic dust and interstellar clouds.” The challenge of “creating an overarching narrative” that united the project’s many pieces often challenged Schettino to step back from the details and think conceptually.
Schettino also notes, “It was cool to see how the data mirrored the physical structure of the instruments; for example, NuSTAR [the Nuclear Spectroscopic Telescope Array] has two focal planes” or detector units, whose X-ray data was stored in “separate arrays that needed to be combined.”
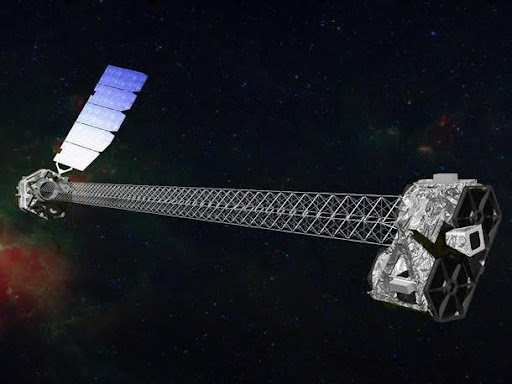
An illustration of NuSTAR. Schettino used X-ray data collected by NuSTAR to help infer a neutrino number for his candidate AGN. Credit: Caltech/NASA JPL.
In the end, Schettino concludes that his summer work “puts into perspective how little I know” in the best way possible; it’s motivated him to keep learning. “There are so many physics that I thought I understood, but I realized I was thinking about them wrong.” In true Bowdoin style, with passion and humility, he remarks, “I’m learning which questions to ask.”