Hot Topic: Professor Rachel Beane Studies Supervolcanoes
By Rebecca Goldfine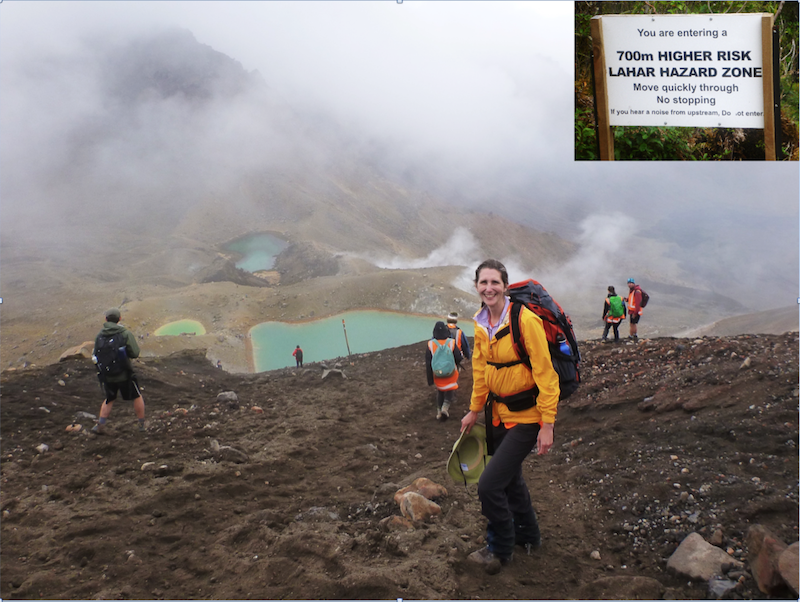
By studying micron-millimeter sized slices of fist-sized rocks collected from the earth’s surface, Professor of Earth and Oceanographic Science Rachel Beane can discern what has been happening miles below ground, in the depths of supervolcanoes. Her investigation of volcanic rocks is pushing forward our understanding of these enormous volcanoes, and could lead one day to better prediction models for their eruptions.
While most volcanoes are scary, supervolcanoes — or “mega-collosals” — are many times worse. Just a handful of places around the world are at some risk for a supervolcano eruption, mainly the Taupo Volcanic Zone in New Zealand, the Bay of Naples and the Aegean Arc.
To get a sense of how big super volcanic eruptions are, it helps to compare them to other eruptions. They exceed the Krakatau cataclysm of 1883, which spewed 25 cubic kilometers of volcanic material, causing global temperatures to fall 1.2 degrees. Temperatures didn’t return to normal for five years. Supervolcanoes also dwarf the 1980 explosion of Mt. St. Helen’s, which ejected 1.2 cubic kilometers of material. In contrast, supervolcanoes emit more than 1,000 cubic kilometers of lava, ash and other material when they blow. They tend to be so much more catastrophic due to the composition of their magma, which is viscous rather than syrupy because of its high silica content. The magma often solidifies before erupting, leading to a buildup of pressure underground that eventually overcomes the resistance. But scientists are still trying to understand the causes of mega-collosal eruptions. Triggers for smaller eruptions are insufficient to generate these massive events.
Fortunately, Beane does not think a supervolcano will erupt any time soon — not that she spends much time dwelling on this scenario. “If I thought about that, the excitement of studying the volcanoes would be gone, because it is potentially horrific what would happen,” she admitted. Beyond the devastation the volcano’s lava and ash would wreak for many miles around the blast, the eruption would push so much ash into the atmosphere that it would likely lower global temperatures for many years, setting off a new ice age.
Last year Beane spent her sabbatical year in New Zealand’s Taupo Volcanic Zone, a highly active tectonic area, looking for clues about the nature of supervolcanoes. While walking in the area, she could sometimes reach her hand down and scoop up handfuls of hot earth, which she described as “wonderfully warming on a cool, rainy day.” While smaller eruptions are not infrequent in the area, the last super volcanic eruption happened in the Taupo area about 27,000 years ago.
Beane and four co-authors recently published a paper in the journal American Mineralogist, sharing the findings from their research in New Zealand. The paper’s lead author is a recent Bowdoin graduate, Karina Graeter ’14, who is now studying glaciology at Dartmouth. The article, selected by the journal as one of the issue’s notable papers, makes the case for a close connection between rocks formed in cooling supervolcano magma chambers and volcanic activity. Magma reservoirs are pools of molten rock 4 to 10 kilometers below the earth’s surface.
With a background in mineralogy rather than volcanology, Beane brings a unique perspective to the study of supervolcanoes. “If we’re going to figure out what might happen in these types of eruptions, we need a different way of looking at them,” she said. “I’m looking at things differently than classically trained volcanologists are…I have learned how to look at minerals and mineral textures closely to interpret big tectonic processes.”
When Beane scours a volcanic area, she looks for plutonic lithics, or fragments of plutonic rocks, which formed when magma cooled and solidified underground. These lithics, and particularly their mineral textures, provide a rare and valuable glimpse into a magma chamber.
While plutonic rocks might hold the answer to supervolcanoes, scientists disagree about the rocks’ origins. One model has it that they form in one large supervolcano magma chamber. As the magma cools and crystalizes, the solid plutons sink to the bottom of the chamber until they are scooped up in a volcanic eruption and ejected. The other model makes the case that volcanic processes and plutonic rocks are only distantly related, and that plutons are formed outside the big magma chamber.
Using high-powered electron microscopes, Beane examines the crystal structure of plutonic lithics, noting characteristics such as how many crystals are clustered together and the orientation of their faces. Last year, she and her team made some interesting observations. The slides revealed that the rocks had undergone one or more rounds of cooling, crystalizing, reheating and melting. “We have a history that shows there have been multiple episodes of resorption,” Beane said. “In other words, the quartz that had initially crystallized partially melted again, then crystalized some more, than partially melted again.” She added, “That process suggests it has been sitting in a magma chamber for a long time. It could be thousands of years or tens of thousands of years.”
Beane’s team also noticed that plutonic lithics contained adjoined clusters of crystals with different histories of heating and cooling. “That suggests to us that in a big magma chamber, different portions of the magma chamber were hot and cold at different times,” Beane said. These clusters then came together in some way that supports a model of a large, long-lived and shallow magma chamber. Supervolcanoes’ explosive potential and the amount of lava and ash they discharge are mostly controlled by processes going on in magma chambers, so the more information scientists have about these pockets, the more they’ll understand the volcano above.
Although there are multiple reheating events during the history of a magma chamber, many of these do not create sufficient melt to lead to an eruption. However, some heating events — most likely associated with a large influx of magma into the chamber from underneath it — do generate sufficient melting that may trigger an eruption, Beane said. She concluded, “I think there is still much to be learned as we study mineral growth and remelting histories from other volcanic calderas.”
Beane’s research was partially funded from Bowdoin’s Porter Sabbatical Fellowship and the Bowdoin Faculty Research Award. Her sabbatical research ties into her volcanic caldera research in Arizona and Colorado, which is funded by an ongoing National Science Foundation grant she received in 2013 (NSF Award 1250259). She was also supported last year by a University of Canterbury Erskine Fellowship.