Professor Dharni Vasudevan Helps Detect Chemical Exposure in the Environment
By Rebecca Goldfine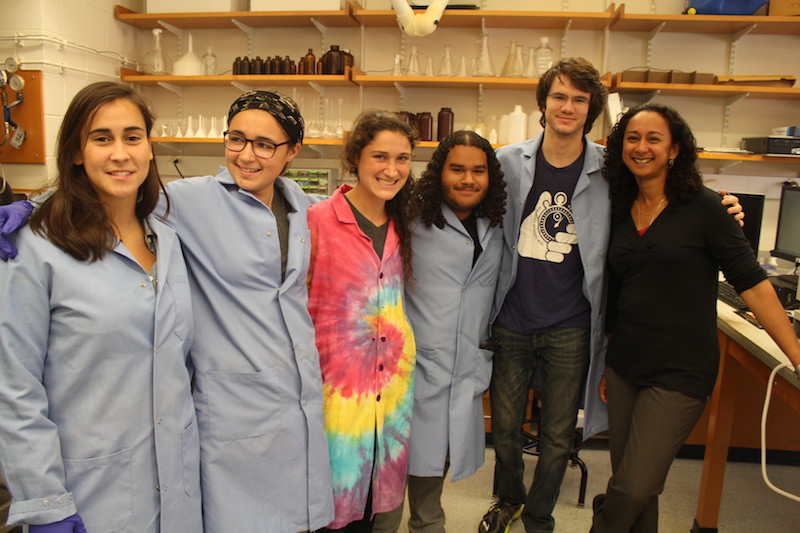
You’re not feeling well, you take some medicine, you feel better. End of story? No. Instead, some proportion of some medicines, or their components, passes through your body and makes their way into the environment, potentially contaminating ecosystems and harming people and wildlife.
Pharmaceutical pollutants derive from a number of sources, not just from people taking pills. Livestock in the United States’ industrialized farming system are administered a huge amount of pharmaceuticals, primarily antibiotics. Aquaculture systems and pharmaceutical manufacturing sites provide other pathways for chemicals to pass into wastewater treatment systems, soil, surface water and groundwater.
To understand the unintended effects of our modern agricultural and pharmaceutical industries, toxicologists and environmental chemists — including Bowdoin Professor of Chemistry and Environmental Studies Dharni Vasudevan — are studying the afterlives of pharmaceuticals and related chemicals. “As an environmental chemist, I’m concerned about the fate of these chemicals in the environment,” Vasudevan said. “And the reason I care about their fate is because I care about exposure risk to humans and ecosystems.”
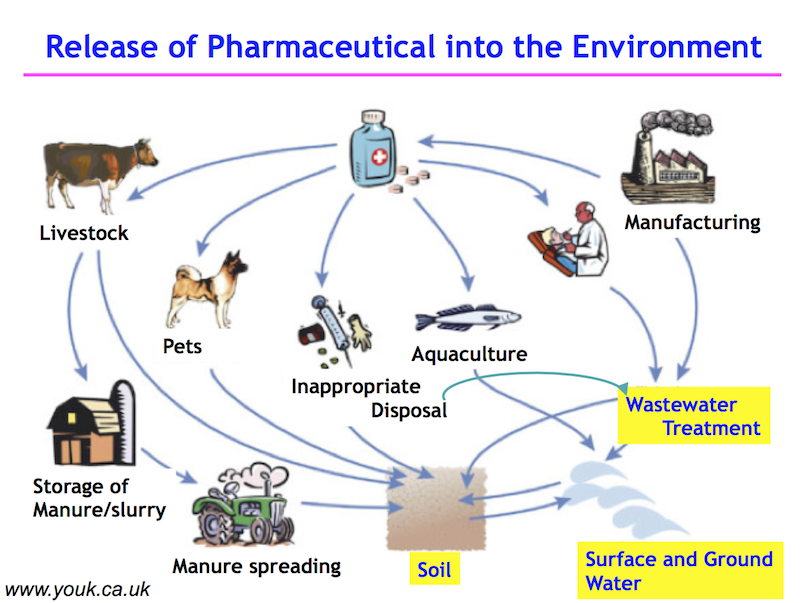
This year, Vasudevan and her research partners at the Ohio State University and University of Connecticut — Allison MacKay and José Gascon — won a National Science Foundation grant to investigate the trajectories, or “fates,” of common medicines — including antibiotics, antidepressants, heart drugs and pain medication.
Vasudevan’s work is part of a new wave of research in her field of environmental chemistry. Before 2000, most environmental chemistry was still focused on an older generation of contaminants, like PCBs, PAHs, DDT and other chlorinated insecticides. But those compounds behave differently than ones used in medicines and personal care products.
Scientists and policy makers have only in the past decade or so began to seriously look at these newer chemicals, their work made easier by the development of more precise detection instruments. In the early 2000s, the U.S. Geological Survey did a large study of the streams and groundwater in the United States, picking up worrisome traces of chemicals that are common in human and veterinary medicine. For example, “we’re finding small quantities of antibiotics in aquatic ecosystems,” Vasudevan said.
This doesn’t necessarily mean people’s health risk is increasing, or that more fish are in danger of becoming sterile. Vasudevan said scientists are trying to find out what exactly is happening,. “What might be the effect [of these contaminants]?” she asked. “Are we at risk? And if we’re at risk, are we exposed? We can be exposed and there is no effect.”
Vasudevan began studying “emerging contaminants” in 2003. In particular, she has been looking at how compounds with positive or negative charges (or both) move from water into soil, or liquid into any solid, a process called sorption, and whether they move from the soil back into water systems.
While some study the possible effects of contaminants on humans, Vasudevan’s expertise is more on the “exposure side of that scenario,” she said. For instance, her work is relevant to understanding whether an antibiotic is building up in the soil and contributing to microbial resistance to that antibiotic. But microbial resistance would be a worst-case scenario. Soil might also be acting like a filter, holding onto contaminants so they don’t pollute groundwater. “Depending on the scenario and the chemical, sorption could be a good or bad thing,” Vasudevan said.
Professor Dharni Vasudevan used some of her NSF grant to hire James Sullivan ’16 as a research assistant. This summer, he began developing a new method for calculating how positively charged compounds, or cations, interact with negatively charged soil components.
To achieve this (and it will be a lengthy undertaking), Sullivan begins with a fairly simple compound and calculates the extent of its sorption into soil (or, the process of moving from water, or from a liquid, into a solid). Next he calculates the sorption of a compound that is very similar except for the addition of a new structural moiety, or piece, in the compound’s chemical structure. Then he looks at the difference between the extent of sorption between the two to advance Vasudevan’s predictive model. “I’m trying to mathematically calculate how changes in structure — or, these additional pieces of a compound — contribute to sorption,” Sullivan said. “The hope is that after I finish my research this year, we can have a lot of the procedures and methods done and demystify the math,” so that future users of the predictive sorption model, such as the EPA, can easily use it, he added.
Sullivan said he appreciated the amount of independence Vasudevan has granted him in the research project. He also credited his professor for helping him develop as a student and researcher. “She’s a tough mentor,” he said. “She expects the best from you.”
Yet to determine how every chemical interacts with every kind of soil would be a herculean task. “The soil in the our home’s back yard is different from the soil in the front yard,” Vasudevan said. “If we wanted to understand the fate of antibiotics in every location, and we did it experimentally, measuring it in the lab, it would be extremely time consuming, expensive and laborious.”
Instead, she and her research partners are building a predictive model based on years of experimental data. They have been conducting, and will continue to conduct, lab tests on a range of interactions. The research is based upon work supported by the National Science Foundation grant CHE-1404459.
The models that Vasudevan and her colleagues are creating will be useful in labs across the country. “We will build quantitative frameworks that the Environmental Protective Agency or any other organization that is looking at exposure risks to humans and ecosystems could use without having to make measurements,” Vasudevan said. Although her research at the moment is focused on pharmaceuticals, the models could be used to learn the fate of other kinds of chemicals, including pesticides and ingredients in personal care products.
Vasudevan said she hopes that her research, and other new research by environmental chemists, could help modernize United States’ chemical policy. “Our laws are outdated,” she said. In comparison, Europe is much more stringent in its regulation of industrial chemicals, and has banned many chemicals that are still legal in the United States.
“[Chemical policy] is based on how societies perceive risk, how precautionary they are,” Vasudevan said. But countries can overreach, she added. “In many cases when Europe bans a chemical earlier than the United States, they could potentially lose out on possible economic benefits. Balance is needed.”